I. INTRODUCTION
The development of modern information technology in the management and automation of technological processes and research is largely based on the establishment and application of effective recording devices and digital signal processing. A typical example of information processing systems with many-element transducers are the primary signal processing system of acoustic emission (AE) [1-5].
Improving methods and means of measuring the acoustic emission signals, the emergence of new principles of control and diagnostics of technical objects with the use of various physical effects leads to the need to create and study more and more advanced mathematical modeling techniques and analysis of signals as the source of the experimental data, with a focus on computer implementation.
II. ANALYSIS OF THE PROBLEM OF DEVELOPMENT OF METHODS AND ALGORITHMS FOR SIGNAL PROCESSING OF ACOUSTIC EMISSIONINTRODUCTION
Analysis of the literature in recent years, in research on the development of methods and algorithms for signal processing of acoustic emission transducers multiple systems control hardware, indicates that achieving significant theoretical and practical results in this area. There are also developed various ways of building high-performance signal processing algorithms in a varying degree of a priori uncertainty. At the same time, there are not fully address issues related to the purpose of developing high-precision methods and algorithms for signal processing transmitters. There are several reasons for this state of affairs in the field of processing encoder signals, the integral effect of which is manifested in poor and poorly controlled as computational processes in information and control systems and data processing systems. To ensure high quality signal processing systems of different nature must be greater involvement of mathematical methods and approaches can significantly improve the accuracy of the signals being processed. This circumstance is an urgent need to address a number of methodological problems of constructing signal processing acoustic emission transducers in systems of non-destructive testing and its application to specific objects of automatic control.
III. INVESTIGATION OF THE OUTPUT SIGNALS OF ACOUSTIC EMISSION TRANSDUCERS
On the basis of the proposed methods, software and algorithmic tools [6-8], a study of the processes of acoustic emission in the elements of metal structures of the alloy AMg-6M and welded joints in the presence of technological defects. Mechanical tests were carried out on a tensile force tensile testing machine ZD-10. Error level measurement voltage is ± 4%. AE-study was carried out using a special meter of acoustic signals. Along with the AE parameters were recorded waveforms. Operating frequency is chosen between 100 and 300 kHz. In this range, the noise voltage at the output does not exceed 35 mV. The minimum detectable displacement amplitude is 10~8mm. The sensitivity of the system converter is preamp (3-4) • 107B/mm. The output pulse of the electrical signal is limited 5,4V that provides a dynamic range of at least 40 dB.
To describe transformed into electro acoustic channel measurement setup used AE signal integrated model
where x(t) - output signal recorded on the screen of a storage oscilloscope; ti - the duration of the pulse AE; y(τ) - a pulse current input to the sensor; g(t - τ) - weight function setting; t - the time of observation.
To determine the waveform, acting on the input of the piezoelectric transducer, to numerically solve the integral equation (1). For this oscillograms determined signal level sampling interval Δτi is not less than 1/2 ·fmax the values in the upper frequency bandwidth fmax = 500 kHz and sample interval Δτ = 1 ms as an array of numbers in a computer.
The frequency spectrum of the output signal Q(f) is determined by the formula , and the spectral density of the output signal Sx (ϖ) was assumed to be |Q(ϖ)|, where ω - angular frequency; ω=2πf. Power spectral density and AE signals were determined using a fast Fourier transform.
III. DISSCUSSION OF THE RESULTS OF FIELD EXPERIMENTS ON THE STUDY OF ACOUSTIC EMISSION IN ALLOYS AND WELDED JOINTS
As follows from the experimental curves superimposed deformation and acoustic activity (fig.1), in the annealed alloy AMg6M acoustic emission begins at voltages order 20-30MPa. AE count rate at the initial stage of loading (to stress σ≈70MPa) is approximately constant and is 7-12imp/s. Extract the tensile stress (σ) at constant load in any of these points leads to the disappearance of 10-15 with AE. AE waveforms registered in the initial stages of loading (up σ≈90MPa) suggest the possibility of short inlet effect (according to equation (1) with a duration ti is not more than 1 ms) of the relaxation pulse is very short with the front edge.
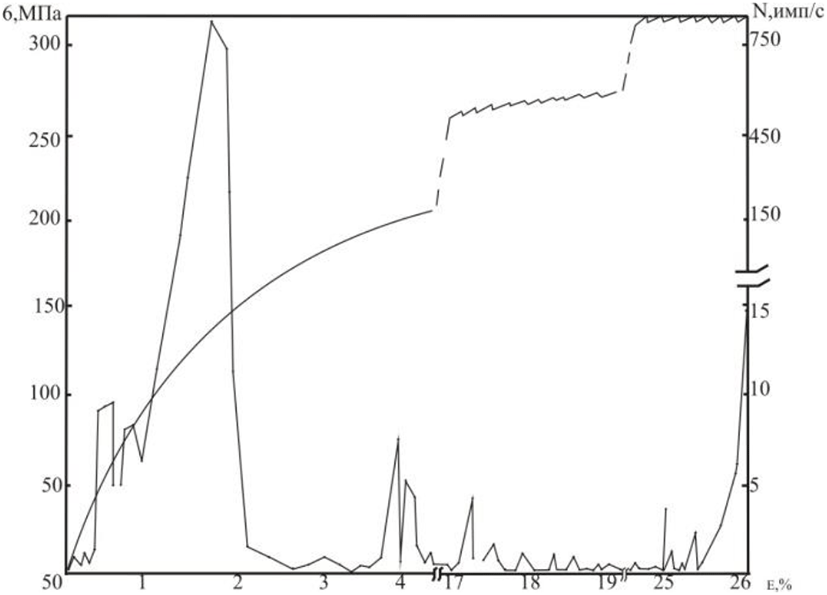
For small tensile stresses AE signals will be similar in form to the impulse response of the sensor, and their amplitude is determined only by the degree of localization of the selected time tp for the elastic energy. Such pulses are the result of the rapid movement of groups of dislocations, which is generated as a result of a collective tear them from pinning points. The amplitude of the AE signals depends on the strength of fastening and detaching dislocations. The frequency spectrum of the output signals AE has the form of narrow-band frequency dependence with a maximum at a frequency determined by the frequency response of the measuring apparatus. Starting with stress 90MPa, the count rate increases sharply and reaches a maximum at stresses σ, close to the physical limit of strength σ = 0,2, 135-170MPa (during deformation ε ≈ 1 ÷ 2 %.).
Such a significant acoustic activity AMg6M alloy in a voltage range corresponding to the yield point, linked apparently with mass education and the motion of dislocations in a significant amount of deformable material. The shape similar to the shape of the output signal of AE (fig.2b), due to the micro fluidity in individual grains (fig.2a), i.e. evidence about the identity of the existing sources.
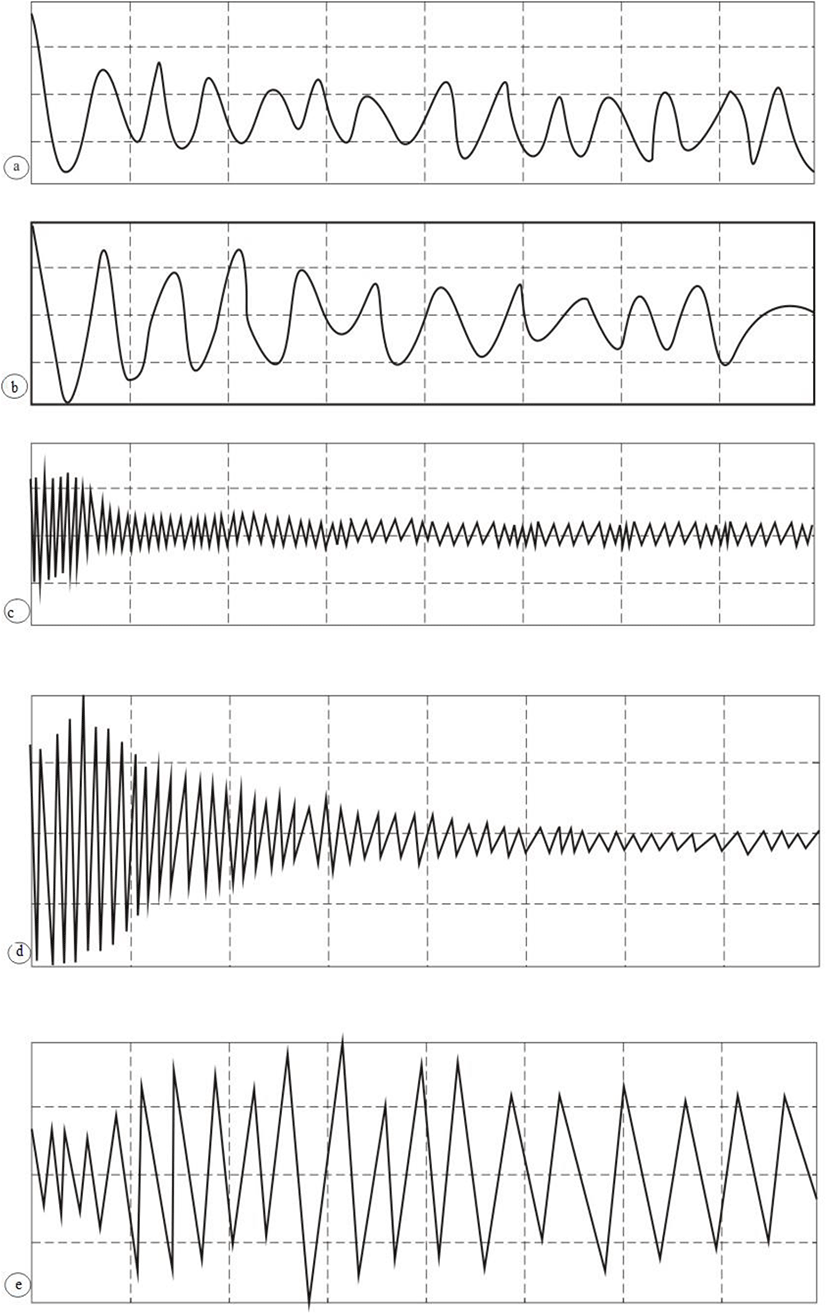
The micro fluidity tensile stresses σ, smaller σ0,2 (i.e. from 90MPa to 35MPa), is due to small oscillations of deformable micro volumes with fast relaxation of stresses in any part of the loaded material occurring in the separation of a large number of dislocations center consolidation. However, AE count rate at voltages of less than 120MPa in order - two less than at σ = σ0,2, constituting a maximum 500-1000 imp/s (sometimes several thousand at a strain rate ε less than 10-3 s-1) .
The amplitude of the AE signals at voltages close σ1,2, small (not more than 400 mV at a gain equal to 8-103 at a frequency of 300 kHz). The spectrum of AE signals in the tensile stresses σ, close and equal σ0,2, similar to the spectrum of white noise with weak low-frequency (40 kHz) part. In a voltage range from 170 to 200MPa acoustic emission is practically not observed (fig.1). A slight increase in the intensity of AE observed in the strain hardening material, since the stress 200…220MPa, and is connected, apparently, with brittle fracture dispersed inclusions and education submicrocracks. Figure 2c) shows the shape of signals appearing in this stage the material damage.
At voltages in excess of 250MPa, the material forms a sub system of microcracks and their continued growth. About this character of damage in the alloy AMg6M says that the output of the Acoustic Signal Meter (ASM)-3 there is a large number of AE signals in the form of a damped sinusoid with a gradually increasing amplitude with increasing load. Some of these signals are not recorded recorder as their duration does not exceed 0.1ms. The spectrum of the pulse spectrum has the features of a polyharmonic process.
For defect-free alloys annealed AMg6M education system of microcracks in the matrix ends at about tensile stress of 280…290MPa, i.e. 80 … 85% σ3. As a result of stress relaxation in the mouth of one of the microcracks, which reached a critical size, the matrix material is allocated a powerful impetus to the background package AE pulses from plastic deformation in the local area damage at its peak. At the final stage of the growth of hopping to a critical size of microscopic cracks degree of localization of the elastic energy released in a short time increases, which increases the amplitude of the output signal. Several high energy radiation pulses similar to that shown in figure 2d) precedes the final fracture of the material. Thus, the destruction of the alloy samples AMg6M thickness 6…8mm observed 2-4 AE powerful momentum. The first impulse of AE appeared at second at 0.88…0.96 σv; third at 0.96 … 0.98 σv.
When loading faultless welds plastic deformation zones are formed are considerably less than in the base metals to be welded semifinished. The value of tensile strength σв tensile ranges 310…350MPa, and yield strength in the range of σ0,2 =250…300МPа. Acoustic activity welds are much weaker than the base metal (fig.3a). Acoustic emission for them at the initial time is virtually nonexistent. A small number of pulses occurring at loads σ<0,5 σv has a very low amplitude and duration, so is not always possible to register them on the recorder and correctly identified as AE pulses rather than the possible noise fluctuations. The first reliable AE signals reliably recorded only at the final stage of loading, i.e. under tensile stress σ<0,7 σv. This is - a few pulses with increasing amplitude appearing with increasing tensile stress. The output waveform (fig. 3e, 3f) is similar to that in the final moment of loading defect-free annealed alloy AMg6M in the formation and development of micro-cracks (fig.2d). The number of AE pulses during this period is very small (on the order of - two lower than the base material). Immediately prior to the destruction of usually fixed one or more of intense bursts energy. In the voltage range 80 … 150MPa acoustic emission in the defect-free welds practically not observed. This weak activity defect-free weld because the large weld cooling rate promote supersaturation α-solid solution of a solid magnesium, whereby the yield strength σ =0,2 increases almost to the tensile strength σv, namely, the plastic deformation material is a source of pulses weight AE
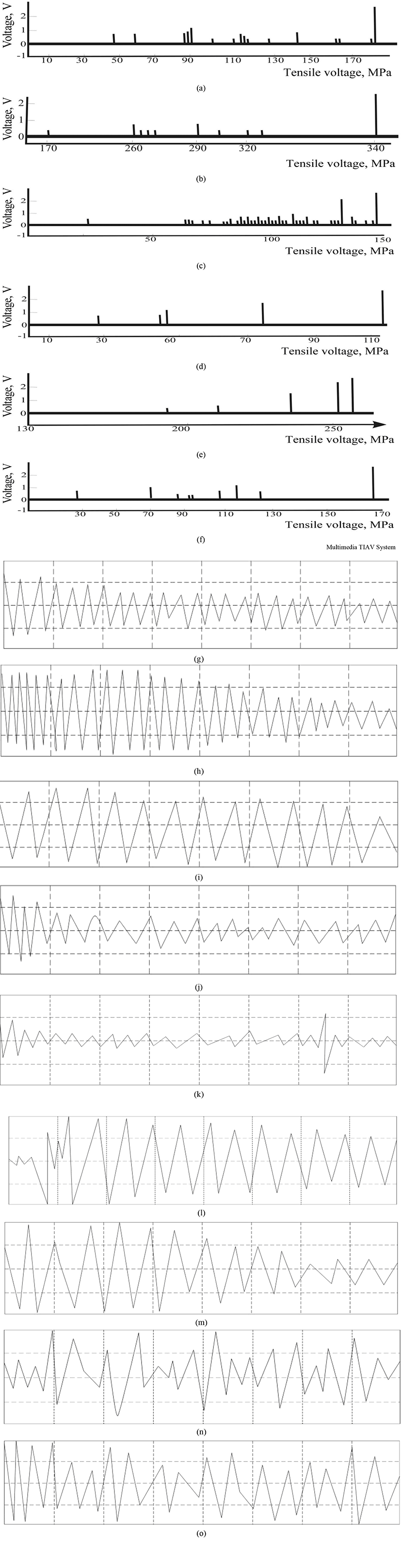
Acoustic activity of the samples weld alloy AMg6M with gas stops permissible according to the technical specifications dimensions virtually identical to the samples of defect-free welds. A similar pattern is observed AE and tensile specimens of welded joints with a pore size is incorrect. However, the larger their size (the greater the influence on the strength of the pores), the pulses appear first before the AE compared to defect-free weld joints (fig.3b). Naturally, the pores are stress concentrators and the more difficult it is to the size and geometry, the faster it is lead to the destruction of the joint. By sporadic large amplitude low frequency pulses and low intensity can be judged without appreciable local volumes in the zone of plastic deformation and then that the source AE in the welds as defects in the pore is blocked in the region of the emergence and development of microcracks in a discrete period of subcritical (fig.3i, 3l). Reliable detection of AE signals from far dangerous size and accompanying oxide captured in a range of tensile stresses up to 160 MPa clearly captures them for later removal.
In samples of welded joints with defects in the form of tungsten inclusions invalid sizes, which are the initiators of the destruction of the weld, acoustic emission is observed for a considerable time sample loading (fig.3c). A weakening of activity AE occurs at the beginning and end of the loading. Obviously, the initial loading time is not involved in the deformation process that creates on the borders of large local stress concentrations. AE source of great acoustic activity NΣ (of the order of 3-10 p/s) with an amplitude of 0.1…1B, apparently, are submicrocrack originating from the sharp edges of stress concentrators - tungsten inclusions.
This assumption is supported by the shape of AE signals (fig. 3j) in the form of a damped oscillation frequency of 2.5 … 3MHz. Such signals are characterized by the formation of the alloy submicrocracks AMg6M and welded joints.
The presence of a stress concentrator in the form of tungsten inclusion leads to an earlier process of formation of submicroscopic and microscopic cracks. Multiple developments of such microcracks, as well as the destruction of inclusions or violation of cohesive bonds at the inclusion of tungsten-matrix weld leads to earlier radiation pulses AE. The smaller size of tungsten inclusions, the later development of the system of microcracks and the less noticeable effect defects have on weld strength. Lack of fusion at the root and at the edge of the seam are dangerous welding defects in the welds are not allowed. If lack of fusion dimensions (length, depth, opening) is much larger than the oxide slivers and cracks, then even at low tensile stress reaches a critical value of the stress intensity factor Kc, leading to unstable growth. The system of microcracks in such samples is not yet formed, and acoustic emissions associated with their formation and development, is absent.
Unstable crack-like defects is characterized by the development of the law of the critical crack length [6]. If the cross section of the material is sufficiently large, the spectrum of critical lengths corresponding to the constancy of the energy per unit length of the crack is observed scale invariance relation previous crack lm length to follow lm+1:
where m = 1, 2, …; Δ - universal constant destruction (for aluminum alloys △ ≌ 0,225); n=2,4,8,…
In terms of destruction - a range of energy per unit length of the crack (or stress intensity factor):
where N - sequence number of the level in the spectrum.
The spectrum Δ1/n has the following form when n = 1,2,4,8,16,32 …, t = 2, 1 = 2.2 mm: 0,225 → 0,47 → 0,688 → 0,829 → 0,91 → 0,95 → … Accordingly, the critical length: 2.2 mm → 4,7 mm → 6,8 mm → 8,2 mm → 9,1 mm → …
Thus, if the thickness of the sample is small (V <8 mm), the crack-like defect depth of about 2mm destroy the sample of the growth of three and will be accompanied by a maximum of three powerful pulses AE. If the size of a large is lack of fusion, the fracture can be occurred in a single leap. Experimental studies confirm these assumptions (Figure. 3 (d) and (l)).
Oxide slivers are distinctive features of development: at the initial stage of their range of AE signals is polyharmonic view, indicating that the action is repeated multiple processes at short intervals of operation. On changes in the amplitude of the curve (fig. 3e, 3f) observed several individual pulses AE occurring long before the destruction (if σ ≌ 0,2 … 0,6 σv). Their form (fig.3m, 3n, 3o) indicates discrete advancements defects. They give rise to the imposition of the output of ASM -3 signals of the “beat” (fig. 3n). Obviously, at the initial moment of the oxide slivers are destroyed her on numerous points of fixing the metal. As a result of the destruction at the site of the oxide formed slivers macrocracks. Its development is in the final stages of loading is no different from a similar lack of penetration or cracks and is accompanied by intense pulses of AE (fig. 3o). Acoustic emission is not registered in the welded joints of the alloy in the range AMg6M tensile stresses 90 … 160MPa, indicates the absence of such seams dangerous for bond strength and crack-volume defects.
The presence of large welds in bulk defects such gas pores, cavities, inclusions tungsten, their chains and leads to the formation of clusters of submicroscopic cracks at an earlier stage of loading than in the case of defect-free welds. AE signals can occur when a tensile stress is lower than 160 MPa, the sooner, the more dangerous for weld defect. These signals are of very low amplitude, short duration (a few microseconds) and the maximum of the spectral density is in the range 1-10 MHz. The total number of pulses AE (NΣ) in welds with such defects determines the rate of formation of the system submicrocracks. For tungsten inclusions NΣ significantly higher than for gas pores.
The advent of powerful AE signals in the range of up to 160 MPa indicates the presence of hazardous weld crack-like defect. AE characteristic of the formation of submicroscopic cracks, missing since the weld has already dangerous defect. To identify differences AE signals from oxide prisoner, cracks, lack of fusion is conveniently performed spectral analysis of signals and determines the power of the output signal. AE signal power from captive oxide, cracks, lack of fusion of small size with increasing tensile stress increases. For oxide prisoner "smearing" of the spectral density of AE signals in a wide frequency range is much greater than for fusions and cracking. The appearance of even single AE signals of high power at low tensile stresses (up to 90MPa) indicates the presence of a dangerous crack-like defect that can ruin a weld in one of the growth of, and demands an immediate remedy it.
IV. CONCLUSION
Studies have shown that information about the form of output signals, and their energy and spectral analysis allow to satisfactorily assessing the nature of the processes of deformation and fracture AMg6M alloy and welded joints. Based on the model, numerous tests, it was found that the relative error of the initial data is δ = 0.005 (0.5%). Were used azimuth, triangulation and dispersion techniques location. From the theory of methods for solving ill-posed problems should, that the relative error in calculating the coordinates of sources of acoustic emission signals in these problems is δ2,/3 = 0.0052/3 = 0,029 or 0.52/3 = 0,629%. The results of the experimental data on the basis of model tests are practically identical with the theoretical values.
Thus, on the basis of research and development to create the appropriate software algorithmic means, the practical realization of the tasks which process the results of field experiment in a production environment on the study of acoustic emission in the alloy AMg6M and its welded joints in the presence of technological defects have shown high efficiency.