I. INTRODUCTION
Hologram visualizations illustrate the unprecedented paradigm of technology that will continue to dramatically affect the medical field. Holographic images are produced by exposing a high-resolution photographic plate near a subject irradiated by a laser or an intense light [1]. The hologram then diffracts light into an image, creating a 3D display of a real-world object. In 1947, Dennis Gabor developed the theory of holography, but failed to further develop his conjectures due to the inadequate light sources available at that time [1]. Throughout the next three decades, researchers and scientists endeavored to find new solutions and methods to advance holographic projection [1]. Perhaps the most significant of these efforts was the “rainbow” holography invented by Dr. Stephen A. Benton. This particular hologram enables multi colored images to be seen under white-light illumination instead of a laser light, which was a vital source before [2].
Although holograms are employed in many different inventions such as the google glass, the oculus, virtual reality (VR), Augmented Reality (AR) and stereo imaging, these projections endeavor to leave a lasting impression on the medical field as well [3]. Endoscopic Holography, the projection of images inside natural cavities of the human body, has enabled researchers to record a 3D high-resolution image of internal organs, allowing a more rapid detection of deficiencies in the human body [3]. Holograms are also now a part of the “surgeon’s tool kit” as they can view the organs of a patient through the screen of a device. Scans that are displayed through holographic images allow surgeons to see bodily defects without actually having to cut open the patient’s body [3]. In addition, different types of holography, such as the external hologram recording endoscope and the multiplexed holography for medical tomography, have evolved as a new class of medical techniques that are not only used for medical diagnosis and detection, but also for industrial testing [3].
Holograms are very dependable, even in emergency situations, as they are difficult to alter or transpose, easily applied on products, and bring forth simpler interfaces and intuitive usages [4]. On the other hand, holograms can be disadvantageous as their production cost is very high, dimensions are limited, and are time consuming when constructing complex images [4]. Although there can be restrictions when using holograms, this paper discusses a particularly efficient usage; creating holograms of brain tumors. By doing this, it can potentially provide many benefits and advancements to surgeons. Although this generation’s technology is exceeding the norm, there is still no technique invented that can allow brain tumors to be fully seen during a removal surgery [5]. The projection of a brain hologram would evidently improve the precision of surgeons because they will be able to see exactly where the brain tumors are located [5]. Because they enable visualization of organs and contrastive structures, holograms will surly bring forth greater assistance in hospitals in the near future.
II. MATERIALS
The brain tumor CT data is that of a patient at the National Cancer Centre in Ilsan, South Korea. The DICOM 3.0 (ISO 12052) tool was used to receive the CT scan that would later be segmented through semi-automatic segmentation. The Analyzer (11.0 ver, Mayo Clinic, USA) tool was used for the process of segmentation through the seed-region method. Through the Region of Interest (ROI) tool, specific image editing was proceeded. After the files were rendered, the Visualization Toolkit (VTK) was used for the image processing and final rendering of the brain tumor image. Finally, LabView 2012 (National Instrument, USA) was utilized to generate holographic frames of the images to later be processed into a hologram. The Holographic pyramid (Shenzhen SMX, China) itself was borrowed from the Chonbuk National University in Jeonju, South Korea.
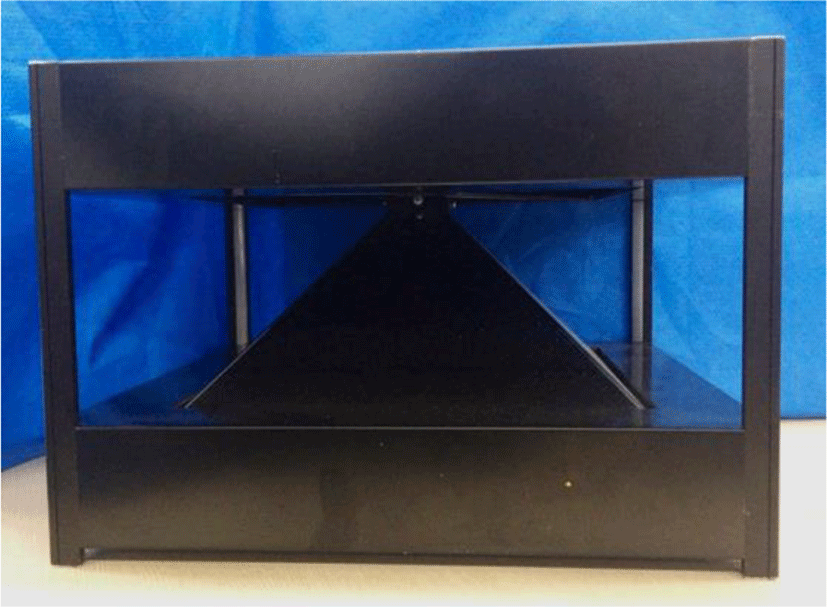
II. METHOD
Digital Imaging and Communication in Medicine (DICOM) is a format that describes a set of operations for transmitting imaging modalities such as Computed Tomography (CT) and Magnetic Resonance (MR) across a network [6]. These messages combine images and metadata to create an effective medical imaging procedure. The DICOM data was used to allow the projection of 3D printing. However, before anything else, the data was segmented into several parts so that it can later be combined together to create a hologram for 3D printing.
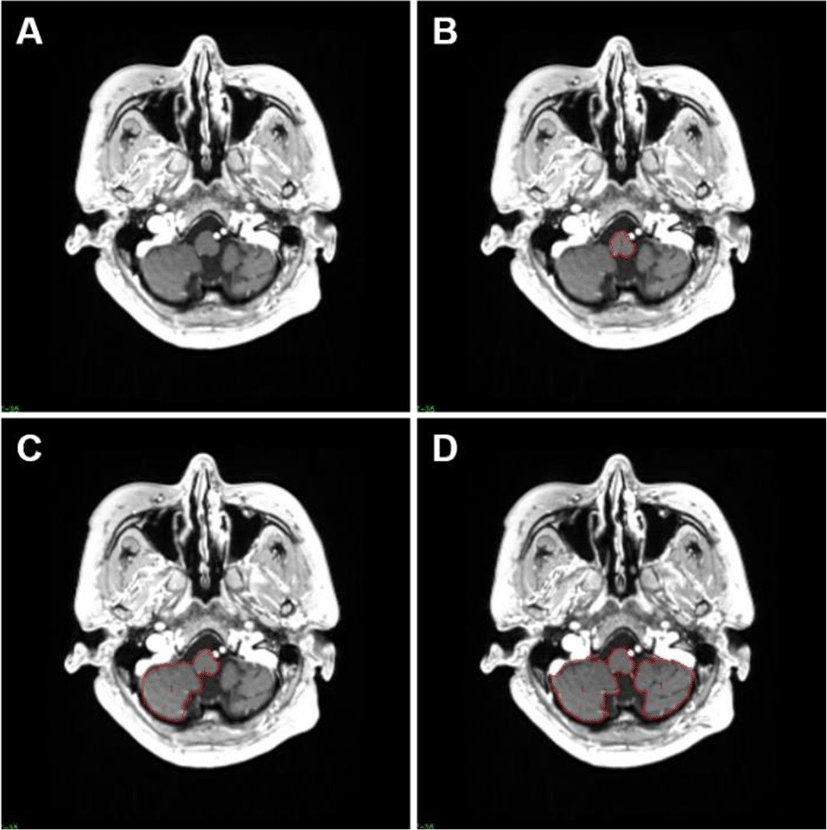
The Region of Interest was traced after the defected area was identified through the specifications of level and width. Through the AUTO TRACE tool, the area of interest was selected. This process was completed semi-automatically as the TRACE tool was also used to manually highlight the specific region. This particular segmentation was carried out through the seed region method. This method starts with a set of “seed” points in a way that the corresponding regions grow by appending to each seed point neighboring hose pixels that have similar properties. Under the supervision of an expert, this process was repeated twice to find the most accurate depiction of the image. It is also possible for both segmentations to mash up to produce the best outcome. The House field unit was then calculated to confirm the visualization of the image [7]. The Window Width (WW) and Window Level (WL) of a specific structure differ depending on the image modality. Because only CT scans were being used in this particular case, the preset (WW-WL) for the brain was 40-80 [8].
Throughout this process, two different files were made: one of the general outlining of the brain and another with only the tumors outlined. The two files were rendered separately, and later mashed together to present an accurate 3D image of a brain tumor.
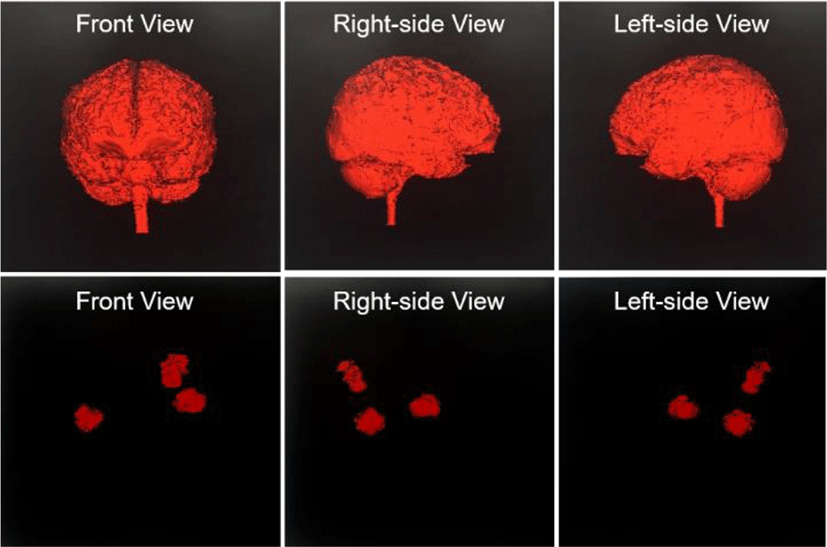
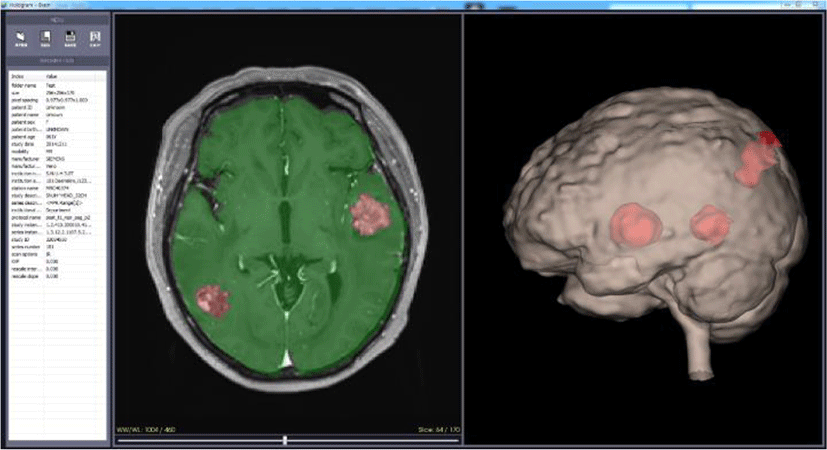
The brain tumor was rendered through a technique called surface rendering. There are two types of rendering: volume rendering and surface rendering. The surface rendering method consists of contouring lines over data points to get an overview of the shape of the object [9]. Volume rendering occurs when the whole structure including the inner parts are rendered using ray casting techniques that allow the ray to be cast from the viewing plane into the object [9]. This technique calculates one individual cell of the image at a time, forcing the process to be slower. Therefore, surface rendering was used because it could be done rapidly and, more importantly, the brain tumors are visible because of the empty inside. The marching cubes algorithm was also utilized in the experiment. Marching cubes creates triangle models of constant density surfaces from 3D medical data [10]. It uses edge intersections to display a polygonal structure that illustrates how the surface cuts through each designated cube displayed in the 3D data set [11].
Data was transferred into holographic data through the LabView2012 (National Instruments, USA) program. There were 360 frames created in total, each frame having been copied three times. Frame “A” was placed on one end and the copied images rotated in the following angles: 90°, 180°, and 270° respectively, were placed on the other three sides. Through this, a 360degree rotation was projected.
After the structure finished rendering, it was projected onto a holographic pyramid through the Pepper’s Ghost method. This optical illusion technique was initially used to create ghostlike figures in performances. It was created by placing a sheet of glass away from the stage between the audience and the actors. An off-stage actor would be lit and reflected by the glass, becoming directly visible to the audience. Similarly, the brain tumor images were placed on four opposite sides of the holographic pyramid. As the projection occurred, all four images were reflected to delude the audience of the existence of an actual hologram.
As shown in Figure 6, the brain tumor image was rotated 1°for each frame and then mashed together to create a video-like product.
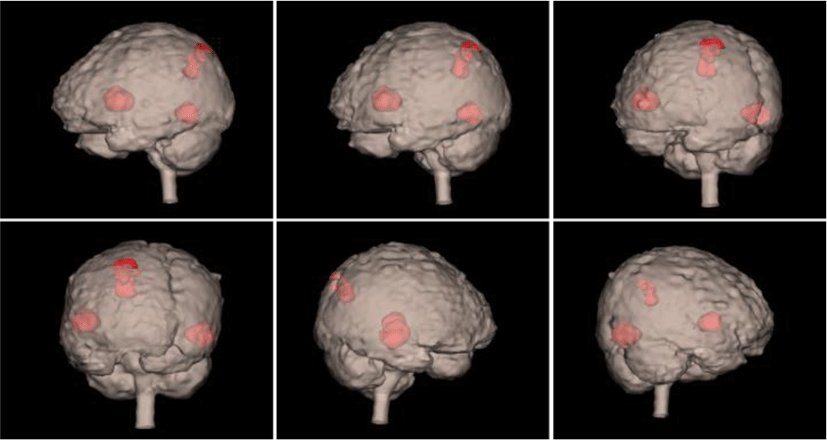
III. RESULTS
Through this experiment, the tumors in a brain were shown more accurately in a 3D visualization. This can be utilized to array defects in one’s body with more exactness allowing experts to rapidly detect something that is abnormal.
Providing3D anatomy visualization allows physicians to comprehend the complex structure and anatomy present that were not visible in the CT scanning. Using methods of auto-segmentation, hologram imaging of a brain tumor was successfully carried out to show the particular ways holograms are utilized in the medical population. We drew the ROI regions of a brain CT scan through auto-segmentation and the seed region method to construct a three-dimensional model of the brain composition with only tumors visible inside it.
IV. DISCUSSION/CONCLUSION
CT scan data was changed into 3D graphic images through the process of semi-automatic segmentation in this experiment. The images were rendered through surface rendering in the procedure. While using the hologram, an individual’s structure was displayed so it was crucial to give the patient the privacy needed by making it anonymous when sharedtwithothers.
Although holographic images of brain tumours can be very helpful, there were still some dissatisfactions felt during the process of experimenting. It took a fairly long time to perform the semi-automatic segmentation, because it was necessary to go through every frame to detect the ROI. Therefore, it would be very advantageous to use a tumour detector such as the CAD (Computer aided diagnosis) as a more rapid alternative.
It would be very useful for surgeons if the brain tumor hologram is registered to be used during the surgery so that the surgery can be done with better precision. The hologram would permit surgeons to see the overall picture of the tumor and where it is in the brain. Having a larger display of the graphic imaging would also be beneficial although it may cause distortion to occur from the light sources. In reality, to use this information in hospitals would require the application of an automated algorithm in software or hardware. The presentation and discussion of many other cases is critical to conclude how holographic imaging can be utilized in the different fields of study.
An advancement in this generation’s technology is also necessary to set the standard for use of holograms in hospitals in the near future. Despite these drawbacks,
Holography imaging will soon allow doctors and researchers to test insertion of medical instruments into a three-dimensional artificial structure before a surgical operation occurs. In addition to the many imprints holographic imaging will leave in the field of medicine, it will also be a boon to machines people use every day such as smart phones and memory devices. Using holography in our banal devices will allow a huge increase of the memory capacity of phones. The potential utilizations of holograms, especially in the area of science, are increasing rapidly. In the next ten years, it is evident that they will become the paradigm of communication in countless different fields of study.