I. INTRODUCTION
The purpose of this paper is to promote mobile and ambulatory communications, especially for ambulances. This paper discusses optical data communication in urban and suburban areas of Japan via fisheye cameras. The approach proposed would permit the recording of simultaneously captured digital data received via the S-band from a mobile communications satellite (NTT DoCoMo’s N-STAR GEO) with an onboard tracking system, based on directional and positioning data obtained by a GPS gyrocompass.
This paper proposes methods, experiments, and a model for obtaining a nationwide communication profile to help predict the propagation of mobile communication satellites and to support ambulatory applications, including forward video transmission links. We emphasize the benefits of deploying HEOs (Figure 1) to address issues associated with obstructions to line-of-sight communications.
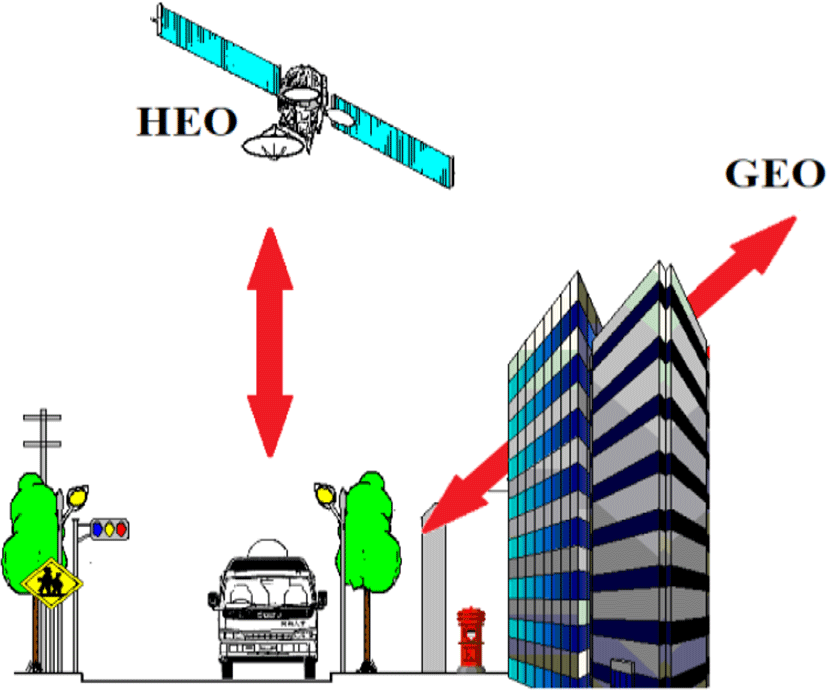
II. BACKGROUND
Propagation effects with the LMSS (Land Mobile Satellite Service) differ from those for fixed satellite service (FSS), primarily due to the greater significance of terrain effects, including trees, buildings, hills, and mountains.
FSS generally allows discrimination against multipathing, shadowing, and obstructions through the use of highly directional line-of-sight antennas placed at unobstructed sites.
The third-generation (3G) mobile communication technologies already introduced in developed nations address certain data transmissions (64 kbps x n) from moving vehicles in urban areas. However, precise observations of patient conditions by clinical physicians depend on high-quality images of 2 Mbps and/or 10 Mbps, with compressed HDTV from ambulances en route. Certain clinical cases require high-quality images to assess the condition of patients in critical condition, such as HDTV compressed with HEVC (High Efficiency Video Coding, or H.265), transmitted from moving ambulances.
The third-generation wireless cellular phone (the 3G wireless mobile phone) is called the 3G phase 1, or Wide-band CDMA in Japan. NTT DoCoMo has already offered full service in 99% of the surface area of Japan. In the transmission of video data from an ambulance to an emergency center, the transmission capability of the upstream line (from the mobile station to the transit node) is of great importance. NTT DoCoMo offers three kinds of upstream line services. As ambulances run at a maximum 80 km/h on the street, the data transmission is to be conducted at a rate of 144 kbps by FDD (Frequency Division Duplex) via macro Node B over a maximum transmission distance of 15 km. It is difficult to conduct upstream transmission at 2 Mbps from a high speed vehicle in an environment in which frequency selective fading will be generated due to multipath interference urban areas.
5G/Ka-band is expected to support significantly faster mobile broadband speeds and higher volumes of data use than previous generations (the latter is based on the LTE, or Long-Term Evolution, standard). The ITU-R standard for wireless broadband communication will expand the functionality of mobile devices and data terminals while unleashing the full potential of the Internet of Things. As a core fiber-through-the-air technology, 5G is expected to support autonomous cars in smart cities and the industrial Internet and play a key role in the future of telecommunications. 5G/Ka-band will also prove essential in maintaining today’s most popular mobile applications, including on-demand video, by ensuring that growing uptake and usage can be sustained.
However, 5G/Ka-band uses wideband frequency resources with 500 MHz bandwidth on 28 GHz. This means the frequency characteristics related to blockage and shadowing are dramatically different from the lower microwave frequencies. Communication distances for 5G/Ka-band will be 100-200m. Since the operating principle is primarily line-of-sight, carriers will need to deploy many repeaters and transponders across the urban landscape.
Since HEO can cover Ka-band obstructions, we expect HEO to prove highly effective in supporting forward video transmission from moving vehicles to emergency triage centers.
III. METHODS
Multipath fading is due to the constructive and destructive combination of randomly delayed, reflected, scattered, and diffracted signal components. Under the multipath environment, there are three models designed mathematically.
The Nakagami-Ricean density distribution is given by formula (1)
Io : Zero-order Bessel function
MrA: average power of scattered wave component
K: Rice coefficient
This propagation environment consists of one strong direct wave (line-of-sight) and weak multiplex propagation waves.
It is assumed that the received signal is affected by nonselective Rice fading with lognormal shadowing on the direct component only, while the diffuse scattered component has constant average power level. It means that a direct wave declines by lognormal distribution caused by leaves of roadside trees. In such propagation environment, a synthetic wave ingredient will serve as Loo distribution.
MrB: average power of scattered wave component
When a line-of-sight signals are influenced by buildings, the direct path is entirely blocked. This propagation condition is referred as deep shadowing state, a synthetic signal is based on stray multipath components which obey Rayleigh distribution.
MrC: average power of scattered wave component
Fading among channel states is a relatively slow process if the vehicle is relatively distant from buildings or trees (for example, 5 to 10 m). This fading is determined by the distance of user movement and elevation change, each state maintaining a certain geometric space. The change in states is described by the Markov chain process. When a mobile terminal moves over a certain distance, it switches randomly to a new state. Figure 2 illustrates modeling for the three-state Markov process. We observe a strong relationship between the three propagations of the fading channel and visibility (optical path) taken by fisheye camera shown in Table 1.
Microwave propagation | Optics | |
Line-of-sight | Ricean fading | Visible------------- |
Shadowing | Loo distribution | |
Blockage | Rayleigh fading | Blockage |
We captured the images recorded by the video system using a computer, and converted them into digital motion pictures. The motion-picture files (in the AVI format) were converted to still images corresponding to each frame (bit map). To enable the easy analysis of images, the color images were converted to monochrome binary (two-level) images (Figure 3) in accordance with a predetermined threshold.
The length from the center to the first black region of the binary image gives the minimum elevation angle in the corresponding direction. On the other hand, direction from the center to the bottom of the image agrees with the running direction. Thus, if this direction is matched with the direction indicated by the GPS compass (the running direction of the vehicle indicated by the data synchronized using the time code and the GPS timer), the running direction of the vehicle can be calculated as an absolute value.
First, we can calculate the satellite position from the (x,y) coordinates of the two-dimensional binary images. To transform the (El, Az) coordinates from the (x,y) coordinates, we have to model geometric correlation shown in the Figure 4. With the following equations, we can calculate the elevation and the azimuth of the target satellite.
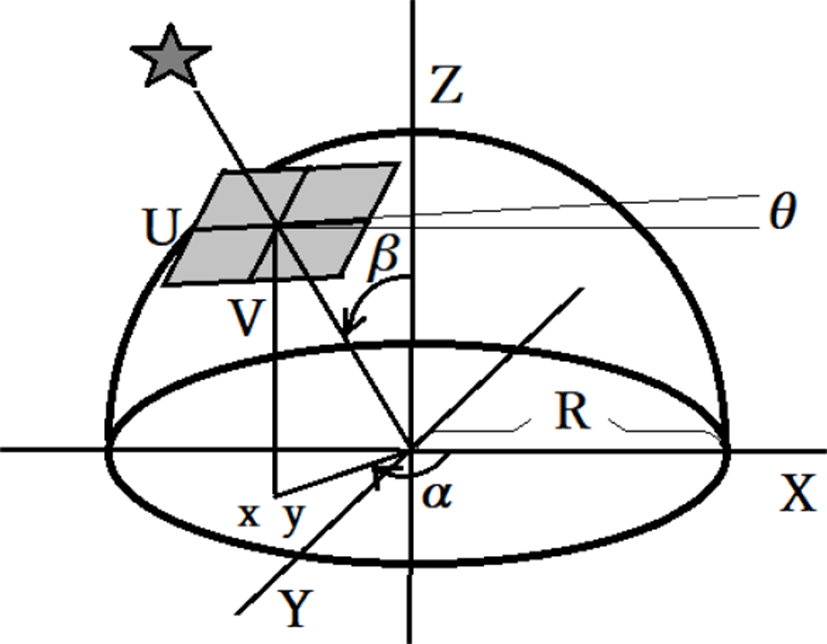
Described above is the concept. Actual implementation of the scheme requires mounting the satellite receiver so as to obtain the beacon signal from N-STAR; deploying a fisheye camera with graphic image recorders; using multichannel analog records for the linear data from the experimental vehicle; leveraging GPS navigation data; and using the direction of vehicle movement detected by the GPS gyro (Figure 5, 6, 7).
We installed the fisheye camera system on the top of the roof of the vehicle. Since the lens (Nikon FC-E8, circular image, focal length of 8mm covering 183 degree) is designed only for COOLPIX video camera, so we have to convert image with C mount adapter. It recorded with the HDTV video recorders (Panasonic DVCPRO 50, 30 flams/sec.) Also, we mounted HDTV video camera to take a front view of the vehicle.
The N-STAR Mark-a satellites will provide fixed and mobile telecommunications services for a design life of over 10 years over the Pacific Ocean. N-STAR Mark-a provided telecommunications coverage for the Japanese Islands and its territorial waters. We used this beacon signal on 2.5010 MHz as a pilot signal, designed a 7.5 tern helical antenna (12dBi, C=0.95; axial type) on S-band with tracking motor (max. angler speed 30deg./sec.) based on the vehicle’s direction data obtained by GPS gyro. The C/N of the beacon signal was over 10dB at the line of sight propagation. However, in case of severe multipath environment at an urban area, we selected the phase composition with 4 helical antennas to detect fine direction of the satellite.
We installed a high-precision Furuno GPS satellite compass (SC-60, accurate +/− 0.6 deg.) on the roof of the vehicle to ensure super heading accuracy. This compass is capable of identifying rapid turns of up to 45 deg./sec. The unique tri-antenna system improves accuracy and reduces the effects of yaw, pitch, and roll.
Since this system outputs information only on the vehicle’s heading, identifying navigation information requires a separate commercial GPS receiver.
We integrated multiple channels data to the hard disk of the PC, such as the optical 3 axis gyroscope (FOG: Fiber Optical Gyro by Japan Aviation Electronic Industry), 3 axis acceleration, the pulse (velocity pulse) from a wheel, steering angle, data of a accelerator, and data of a brake. The sampling rate of the A/D converter for the FOG was 15/sec. with 12 bits. This speed is based on the characteristic of FOG for public application recommended by the COCOM (Coordinating Committee for Multilateral Export Controls) and is not the limit speed of an A/D conversion.
The position data, date and time, heading, and speed, provided by the GPS satellite compass were superimposed on the images of the front and zenith views captured by the fisheye camera.
The vehicle heading, speed, acceleration, navigation data, and date/time were recorded on the hard disk of the vehicle orientation measurement system. The time code for the digital video camera was matched to GPS time, and the video camera was synchronized with the vehicle orientation measurement system with reference to GPS time. The two video systems mutually synchronized based on the common time code.
We gathered field data based on travel over a total distance of approximately 10,000 km (Table 2, Figure 8).
In Table 2, the distances are acquired from the tachometer of the vehicle, also showed the elevation angle to N-STAR, average value of visibility to the South. It is very difficult to find out the optical feature from this average value to the South in each city. In common sense judgment, if location was north, a value will be low, if road width was wide, a value will be high. Then, investigation and analysis were conducted in detail to reflect from the spatial city feature more, such as the northernmost town or most crowded shopping area.
Here we discuss the basic field data analysis in items A, B, and C; the characteristics of Japan’s northernmost city in item D; and the largest urban area in item E.
Figure 9 shows the minimum elevation angles observed on the south side (180 degrees) during an east-to-west drive in the suburbs. In contrast, a drive in the 45-degree direction, i.e., northeast, showed virtually no obstacles. The transverse axis represents the number of frames (distance = velocity × time). The obstacles that periodically interfere with optical visibility are utility poles.
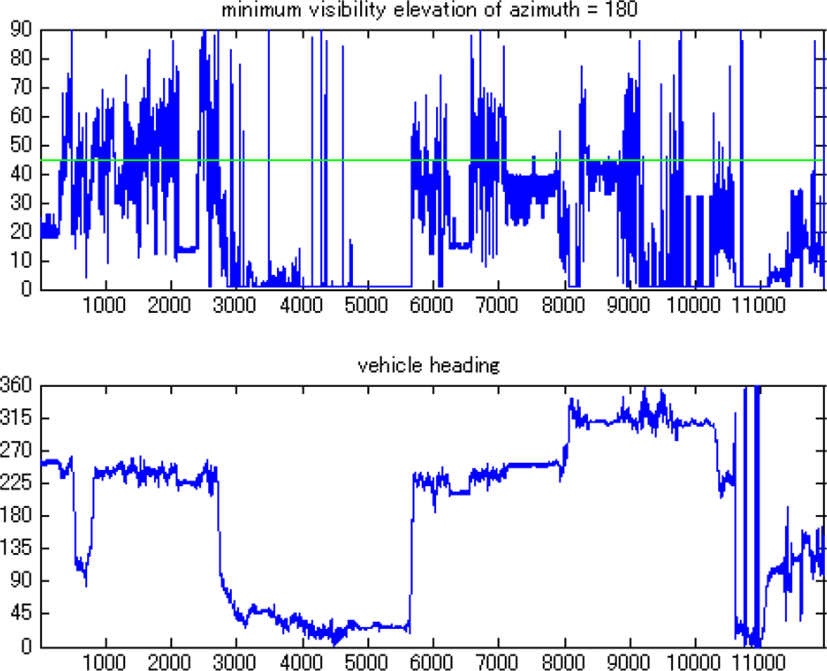
In order to confirm the Loo distribution and multipath effect on the S-band, we drove the experiment vehicle slowly along a road near a building and recorded the angle of elevation and the voltage of the received signal from the satellite (Figure 10).
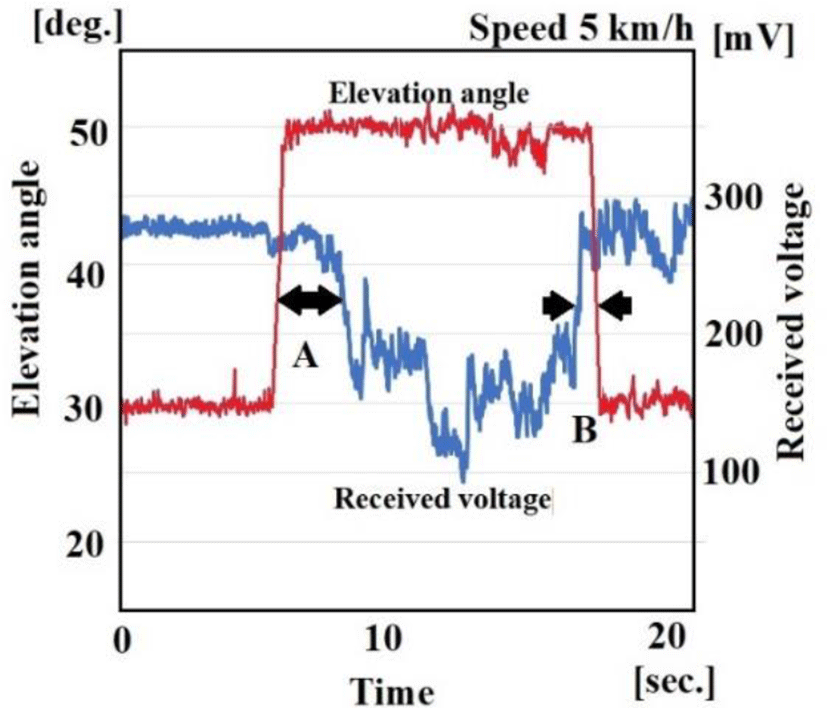
We encountered two types of time lags, A and B, between the elevation angle and the voltage of the received signal. It is reasonable to suspect reflective waves (multipathing) will affect A more than B, and that this multipathing is due to differences in geometric structure from building to building and/or the direction to the satellite. We accept that errors will result when applying optical measurements to microwaves at low frequencies like the S-band. These errors will shrink with higher frequencies.
Meanwhile, obstacles appear continuously 30–40 degrees during the drive in the downtown area. These are buildings (single-family homes in the traditional Japanese style). We plotted the data obtained to show the cumulative frequency of obstacle lengths in Figure 11 for the downtown area.
The distance of the blockage becomes 2.3 m at an angle of elevation of 60 degrees. The most common obstacles blocking optical line-of-sight are utility poles of less than 5 m in height. Both in the suburbs and downtown, all obstacles other than pedestrian bridges were less than 2 m in height. Thus, the spatial diversity (width of the base between the two antennas ) on the vehicle must be at least 233 cm.
Wakkanai lies in the extreme north of Japan, located just 40 km from the island of Sakhalin. The angle of elevation to N-STAR Mark-a is just 36.8 degree. Thus, we investigated the angle of elevation and blockages. Figure 12 shows the route in Hokkaido, including Wakkanai.
With Japanese road environments in mind, we analyzed the relationship between visibility and azimuth angle to the south. We drove the vehicle on the test courses in downtown Wakkanai, including those extending in different directions at 45-degree intervals to 360 degrees. Here, we calculated the cumulative angle of elevation and visibility as percentage values based on a test drive over a relatively narrow road in downtown Wakkanai. Since cars proceed on the left in Japan and approaching buildings therefore lie to the left, Figure 13 can be interpreted to indicate the significant obstruction to the south caused by built structures.
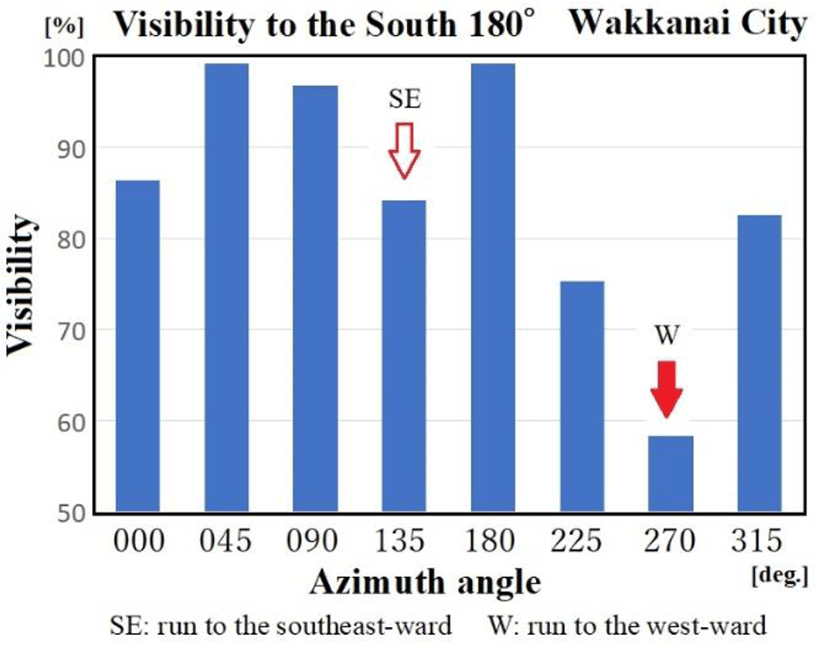
Ginza is currently regarded as the most densely built area in Japan. The angle of elevation to N-STAR Mark-a is 48.2 degrees. Ginza is characterized by numerous narrow alleys connected to main streets (in red or blue; Figure 14)
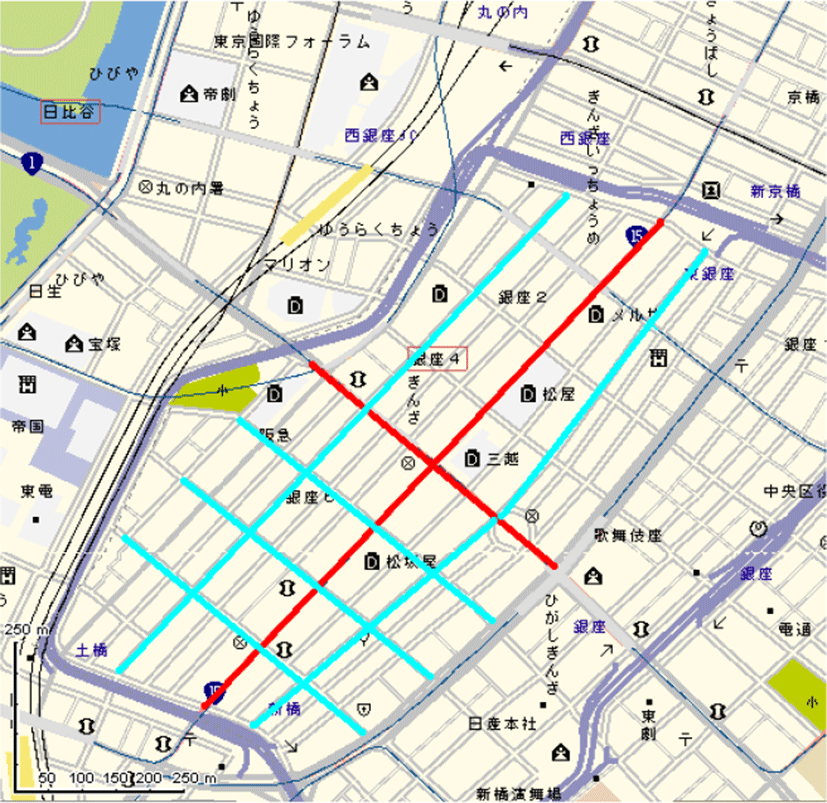
Here, we report on an analysis of visibility to the south (azimuth 180 degrees). Figure 15 gives overall averages, Figure 16 data for alleys, and Figure 17 data for main streets. In each figure, black represents actual field data, dark gray is urban, and light gray is suburban simulated by Prof. Yoshio Karasawa’s Formula (11) published in IEEE Trans. VT[06] .
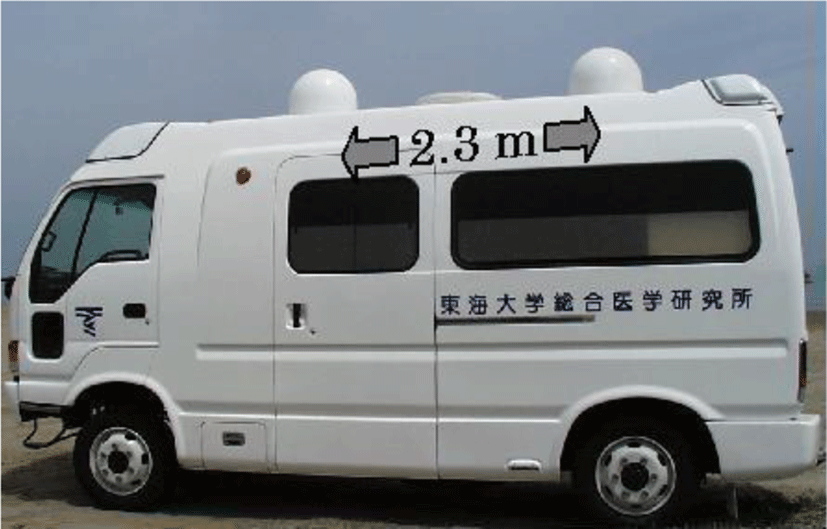
Based on this result, the curve from 20 to 50 degrees is almost the same as Karasawa’s expectation, and the alleys in Ginza have severe blockages from 10 to 50 degrees. For these reasons, we anticipate HEOs will improve performance near these obstructions and enhance 5G communication for moving platforms in Japan’s urban centers.
IV. CONSIDERRATIONS
Optical measurement can be a highly effective way to detect obstacles in urban environments, assuming the method presented here is applied along with a method for recognizing differences based on the blockage of microwave propagation. All obstacles other than overpasses and tunnels do not exceed 2.3 m in distance.
Thus, we determined the optimal spatial diversity for ambulatory applications to be 2.3 m. Prof. Kitano has pointed out the benefits of using a diversity antenna to track two satellites (GEO) published IEEE Trans. AES [07].
Although Russia’s MOLNIYA orbit (12 hours) or TUNDRA orbit (24 hours) have provided practical service for years in high-latitude areas, the inclination angles involved are almost 63.4 degrees. The Earth is not a perfect sphere; its oblate shape generates HEO anomalies. The formula for these fluctuations is called J2; assuming a minimum, we choose an angle of inclination of 63.4 degrees (Figures 19 and 20).
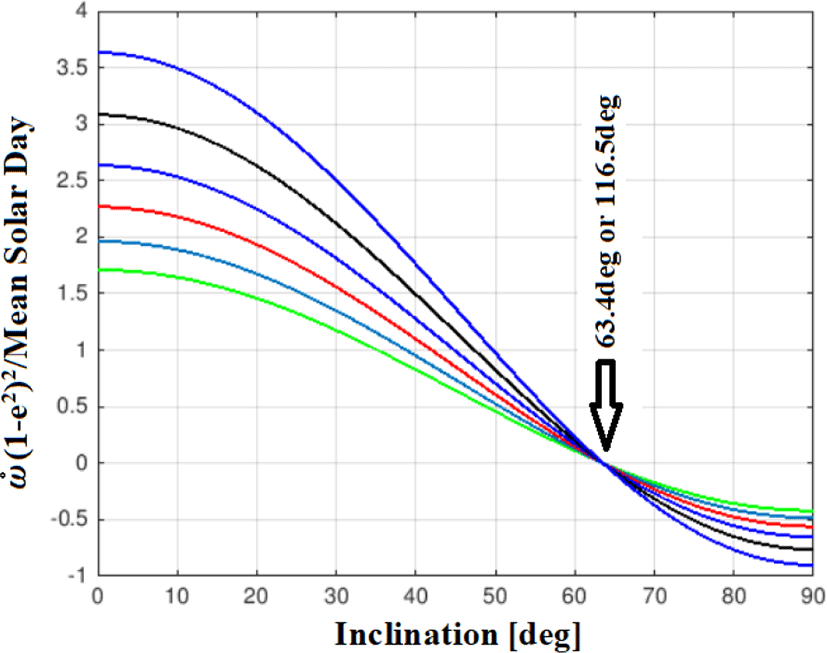
The TUNDRA orbit features significant eccentricity and an extended ellipse. Since the perigee is lower, the Tundra orbit must cross the Van Allen belt twice, resulting in degradations of solar array panels. Thus, we seek a lower eccentricity allowing passage over the Van Allen belt even at perigee. Figure 21 shows the orbital simulation calculated with MATLAB Oribitus ED. In this case, the height of the perigee is 23,137 km, apogee 42,164 km, inclination 40 degrees, and eccentricity 0.3. This orbit would serve all of Japan. The J2 factor of external force from the oblateness is improved (1/1,000 times smaller) than the original TUNDRA orbiter (inclination of 63.4 degrees). The calculated service duration over the angle of elevation 70 degree would cover Wakkanai for 450 minutes and Tokyo, Nagoya, and Osaka for 540 min./one(1) HEO (Figure 22). The minimum elevation angle with four (4) HEOs system shown in Figure 23. For Wakkanai, the figure improves from 37 with N-STAR GEO to 75 degrees.
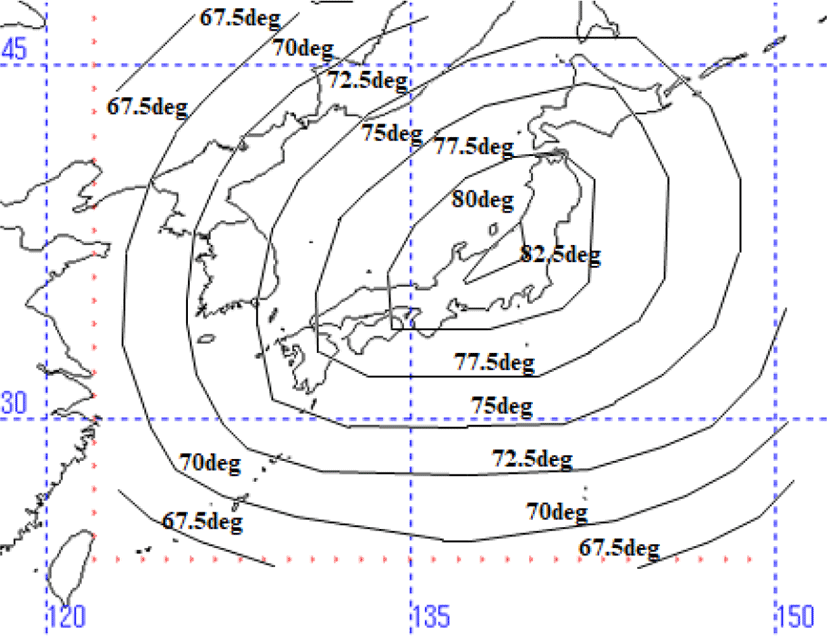
Assuming four HEOs launched with Constellation shifted 6 hours, an angle of elevation by the average value of 24 hours of Tokyo, Nagoya, and Osaka will exceed 80 degrees.
Concerning about forward linkage of video transmission from moving vehicles to a center, this operation condition must be connected a broadband circuit continuously such as 5G/Ka-band and/or HEOs. 5G/Ka-band uses 28 GHz. This means the frequency characteristics related to blockage and shadowing are dramatically different from the lower microwave frequencies. Communication distances for 5G/Ka-band will be 100-200m. Since the operating principle is primarily line-of-sight, carriers will need to deploy many repeaters and transponders across the urban landscape. In case of 5G/Ka-band, it is predicted that short break in the frame level occurs through repeaters and transponders. We are expecting combination operations with HEOs and 5G/Ka-band to solve this problem, because HEOs can keep line-of-sight propagation with high elevation angle for long duration. In such operations, the communications profile on the vehicle based on actual optical data will be very useful to predict blockages and to select/switch a suitable circuit.
V. CONCLUSIONS
Visibility to a satellite is easily determined by checking the value of the pixels in the binarized fisheye image of its position. The process of determining visible satellites is performed automatically. Although surely there is a difference from an optical course and microwave path by multipass (reflection) and/or Loo distribution, our field results are nearly identical to those given by Karasawa’s formula. The launch and introduction of HEOs would significantly alleviate issues related to obstructions in urban areas in the north of Japan (e.g., Wakkanai) and densely urbanized areas like Ginza in Tokyo.
Here we suggest that HEOs could replace 5G in rural areas where 5G is unavailable. In addition, we show that mobile communication profiles based on actual optical data collection can be useful in predicting line-of-sight propagation and/or signal losses for 5G service.